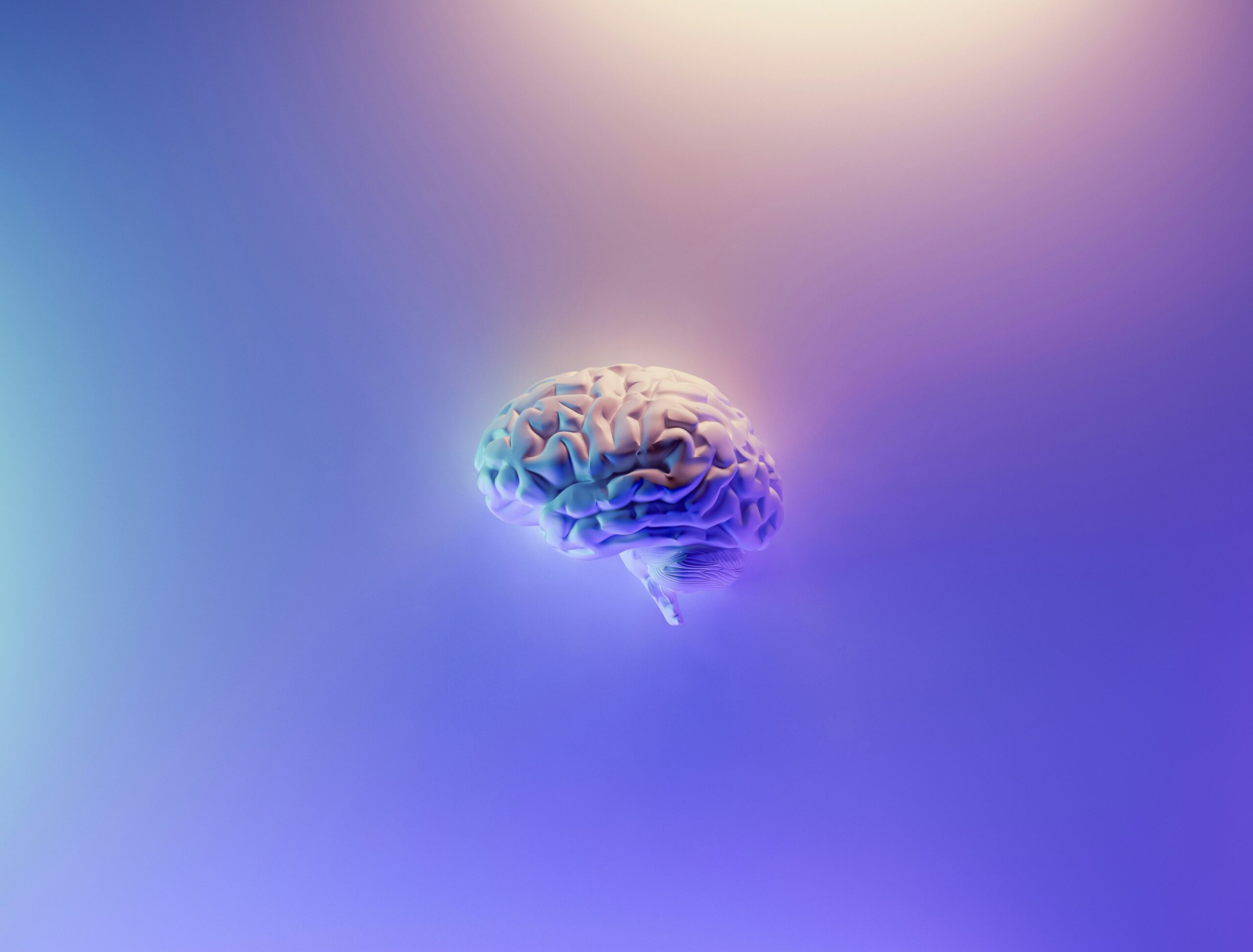
Is it possible?
Would it be possible to create a human brain in the laboratory? Some recent scientific works begin to answer this question.
The brain is the most complex and the most fascinating organ of the human body. Even though it has been the subject of intensive research over the last decades, many areas of its functioning remain unknown, especially regarding the origin and development of diseases. Unfortunately, for reasons too evident to mention, the study of a living human brain quickly reaches multiple ethical red lights.
A little history
To gain knowledge on brain composition and development, biologists had to make up indirect study techniques. Scientists developed the idea of creating organs in vitro in the early 20th century. The discovery of embryonic stem cells in 19811 accelerated the development of the field. Stem cells are a type of cell virtually capable of becoming any cell type of the body when put in appropriate conditions. By extension, it is feasible to create an entire organ by using them. In 2001, a scientific breakthrough led to culturing neuronal precursors made after human embryonic stem cells2,3. However, this technique required decades of effort and the culture of neurons remains tricky: indeed, neurons need many complex interactions with a variety of other cell types to exhibit their physiological behaviour. In addition, mature neurons do not divide, making it impossible to maintain them in culture for a long time. Still, this technological development has made it possible to study, among other things: drug-neuron interactions, neuronal development, and developmental anomalies.
Despite their many applications, there are significant limitations. Indeed, cell cultures contain often only one cell type, sensory neurons for example4. Even if good progresses have been made, it remains rare to have more than three cell types in the same culture. Either way, they lack the complexity of brain tissue composed of many cell types. Moreover, the special organisation of cells and their position relative to each other is an important part of their dynamic which could not be represented in a 2D culture.
In this context, the possibility of having a complete brain in a petri dish is very exciting.
Potential solutions
In 20075, a new technique led to an acceleration of the field. This technique allows reprogramming any mature cell into a stem cell while avoiding the ethical issue raised by using human embryonic cells. In 2005, a group of scientists demonstrated6 the establishment of a 3D culture of neurons mirroring the layering structure of the brain. In 2013, another team revolutionised the world of research by establishing 3D cultures in which neurons had differentiated and self-organised to recreate different brain regions7. These 3D cultures were called cortical organoids, cerebral organoids, or abusively, “mini-brains”. Although helpful in the study of specific cell interactions8,9, or the direct effect of drugs on their biology10,11, such cerebral organoids can only give a partial picture as they are not integrated into a whole organism. Indeed, complex as they are, these organoids are still simple compared with a mature brain. They have no white matter fibres to allow communication between the different areas and are limited by their small size (they are no larger than a few millimetres). Also, in normal conditions, the brain has many interactions with other parts of the body, as well as with the immune and vascular systems. To overcome these limitations, scientists hypothesized that grafting organoids into a mature brain would favour the development of a more complete architecture.
In a recent paper12, the author bet that the grafting of cerebral organoids into an immature brain would help them to integrate and grow inside the host. To test their hypothesis, they transplanted organoids derived from human cells into a postnatal rat brain. Most organoids successfully engrafted into the rat brain. Indeed, they exhibited signs of good vitality such as the development of many connections with both human and rat cells. Moreover, most of the cells had become mature neurons owning an electric activity. This discovery led the authors to question the capacity of these neurons to respond to stimuli and activate some basic rat brain functions. To test this possibility, they touched the rat whiskers while looking at the human neuron’s activity. They observed that the human neurons exhibited electric activity in response to the stimulation. Then, they trained the human neurons to influence the rat’s behaviour: the rat was rewarded with water if it licked during the excitation of human neurons. After 15 days of training, the rats exhibited an increased licking behaviour while human neurons were excited.
Although the results of this study are interesting, there is some room for improvement. Despite the high rate of cell grafting and their capacity to create new connections, the typical layering of the brain was not observed. Moreover, the organoids pushed away the rat tissue instead of integrating the pre-existing tissue into their structure. Thus, their integration was incomplete and needs to be improved for studying complex brain functions. Furthermore, the cells present in the organoids were not representative of the brain cell variety. The addition of immune cells could improve the correctness of this model and allow the study of diseases involving the immune system.
The results of this study open new perspectives in the field of neurodevelopment and medical research. The ability of organoids to integrate sufficiently to impact rats at the behavioural level means that this technique could be used to better understand certain brain diseases, such as Parkinson’s disease or schizophrenia13,14. Indeed, it would be possible to create organoids from patient tissue to observe the development of the disease more closely. This could also accelerate the development of more personalised medicine. By testing drugs on organoids from patients, it would be possible to select the most effective drug for each patient on a case-by-case basis. In the long term, we could even dream of using them to replace damaged areas of the brain, after an accident or a stroke for example.
Ethics, limitations, and the future
Nevertheless, several ethical issues are raised by this technology. The most obvious is the use and sacrifice of animals. Given the growing concerns about the use of animals in research, it is unlikely that this transplant approach will be the future of neurosciences. However, various approaches are being developed in parallel, including the so-called “organ-on-a-chip” approach15-17, which allows cerebral organoids to be grown on a chip mimicking a conventional vasculature and interactions between neuronal tissue and other types of tissues18. This approach avoids another potential ethical problem, namely the possible development of ‘consciousness’ in chimeras with human brain parts. Although this may be the primary concern of many people, this possibility is not yet seriously considered by the scientific community because it is not considered credible in the current state of science. It is critical to not make an abusive link between the reproduction of brain areas, even extended, and notions such as personality or consciousness19. The notion of consciousness is a complex concept to define and study already in the human species so extending it to other animal species seems difficult at present. Not to mention the fact that the transplant technique currently only works with simple organoids, not replacing the entire original animal brain. The idea of a rat with a human brain and mind is still (fortunately) science fiction!
Another problem, less apparent at first sight, is that of the ownership of organoids and the consent of individuals. Some people may agree to donate their cells for research without knowing what the actual use will be. It is common for people to donate their cells or genetic information for altruistic purposes, to help medical research, without being aware that some pharmaceutical companies may then use them for profit20. Moreover, in the context of the creation of a complete organ, the question of the sale of human organs arises. Will the artificial origin of these organs be considered sufficient to justify their trade? Or will the same laws as those applied to organ trafficking have to be considered?
Although this technology is still immature, some scientists have already taken up these ethical questions21,22. The aim is to ensure that we can exploit all the possible benefits of the use of brain organoids while avoiding its abuses.
Sources
- Martin GR. Isolation of a pluripotent cell line from early mouse embryos cultured in medium conditioned by teratocarcinoma stem cells. Proc Natl Acad Sci U S A. 1981;78(12):7634-7638. doi:10.1073/pnas.78.12.7634.
- Reubinoff, B., Itsykson, P., Turetsky, T. et al. Neural progenitors from human embryonic stem cells. Nat Biotechnol 19, 1134–1140 (2001). https://doi.org/10.1038/nbt1201-1134.
- Zhang, SC., Wernig, M., Duncan, I. et al. In vitro differentiation of transplantable neural precursors from human embryonic stem cells. Nat Biotechnol 19, 1129–1133 (2001). https://doi.org/10.1038/nbt1201-1129.
- Wong NK, Yip SP, Huang CL. Establishing Functional Retina in a Dish: Progress and Promises of Induced Pluripotent Stem Cell-Based Retinal Neuron Differentiation. Int J Mol Sci. 2023;24(17):13652. Published 2023 Sep 4. doi:10.3390/ijms241713652.
- Yu J, Vodyanik MA, Smuga-Otto K, Antosiewicz-Bourget J, Frane JL, Tian S, Nie J, Jonsdottir GA, Ruotti V, Stewart R, Slukvin II, Thomson JA. Induced pluripotent stem cell lines derived from human somatic cells. Science. 2007 Dec 21;318(5858):1917-20. doi: 10.1126/science.1151526. Epub 2007 Nov 20. PMID: 18029452.
- Gaboyard S, Chabbert C, Travo C, et al. Three-dimensional culture of newborn rat utricle using an extracellular matrix promotes formation of a cyst. Neuroscience. 2005;133(1):253-265. doi:10.1016/j.neuroscience.2005.02.011.
- Lancaster MA, Renner M, Martin CA, Wenzel D, Bicknell LS, Hurles ME, Homfray T, Penninger JM, Jackson AP, Knoblich JA. Cerebral organoids model human brain development and microcephaly. Nature. 2013 Sep 19;501(7467):373-9. doi: 10.1038/nature12517. Epub 2013 Aug 28. PMID: 23995685; PMCID: PMC3817409.
- De Kleijn KMA, Zuure WA, Straasheijm KR, et al. Human cortical spheroids with a high diversity of innately developing brain cell types. Stem Cell Res Ther. 2023;14(1):50. Published 2023 Mar 23. doi:10.1186/s13287-023-03261-3.
- van der Molen T, Spaeth A, Chini M, et al. Protosequences in human cortical organoids model intrinsic states in the developing cortex. Preprint. bioRxiv. 2023;2023.12.29.573646. Published 2023 Dec 30. doi:10.1101/2023.12.29.573646.
- Ma X, Wang Q, Li G, Li H, Xu S, Pang D. Cancer organoids: A platform in basic and translational research. Genes Dis. 2023;11(2):614-632. Published 2023 Apr 12. doi:10.1016/j.gendis.2023.02.052.
- Zhao H, Yan F. Retinal Organoids: A Next-Generation Platform for High-Throughput Drug Discovery. Stem Cell Rev Rep. 2024;20(2):495-508. Doi:10.1007/s12015-023-10661-8.
- Revah O, Gore F, Kelley KW, Andersen J, Sakai N, Chen X, Li MY, Birey F, Yang X, Saw NL, Baker SW, Amin ND, Kulkarni S, Mudipalli R, Cui B, Nishino S, Grant GA, Knowles JK, Shamloo M, Huguenard JR, Deisseroth K, Pașca SP. Maturation and circuit integration of transplanted human cortical organoids. Nature. 2022 Oct;610(7931):319-326. doi: 10.1038/s41586-022-05277-w. Epub 2022 Oct 12. PMID: 36224417; PMCID: PMC9556304.
- Kim D, Park J, Park HC, et al. Establishment of tumor microenvironment-preserving organoid model from patients with intracranial meningioma. Cancer Cell Int. 2024;24(1):36. Published 2024 Jan 18. doi:10.1186/s12935-024-03225-4.
- Stankovic I, Notaras M, Wolujewicz P, et al. Schizophrenia endothelial cells exhibit higher permeability and altered angiogenesis patterns in patient-derived organoids. Transl Psychiatry. 2024;14(1):53. Published 2024 Jan 23. doi:10.1038/s41398-024-02740-2.
- Wevers NR, De Vries HE. Microfluidic models of the neurovascular unit: a translational view. Fluids Barriers CNS. 2023;20(1):86. Published 2023 Nov 27. doi:10.1186/s12987-023-00490-9.
- Buentello DC, Garcia-Corral M, Trujillo-de Santiago G, Alvarez MM. Neuron(s)-on-a-Chip: A Review of the Design and Use of Microfluidic Systems for Neural Tissue Culture. IEEE Rev Biomed Eng. 2024;17:243-263. Doi:10.1109/RBME.2022.3217486.
- Saglam-Metiner P, Duran E, Sabour-Takanlou L, Biray-Avci C, Yesil-Celiktas O. Differentiation of Neurons, Astrocytes, Oligodendrocytes and Microglia From Human Induced Pluripotent Stem Cells to Form Neural Tissue-On-Chip: A Neuroinflammation Model to Evaluate the Therapeutic Potential of Extracellular Vesicles Derived from Mesenchymal Stem Cells. Stem Cell Rev Rep. 2024;20(1):413-436. doi:10.1007/s12015-023-10645-8.
- Baldwin L, Jones EJ, Iles A, et al. Development of a dual-flow tissue perfusion device for modeling the gastrointestinal tract-brain axis. Biomicrofluidics. 2023;17(5):054104. Published 2023 Oct 11. doi:10.1063/5.0168953.
- van Till SAL, Bunnik EM. The End of Personification: The Mereological Fallacy in Science Communication on Brain Organoids. Am J Bioeth. 2024 Jan;24(1):51-54. doi: 10.1080/15265161.2023.2278564. Epub 2024 Jan 18. PMID: 38236882; PMCID: PMC10805020.
- https://www.edqm.eu/documents/52006/0/OTC-CD-P-TO-Position-statement-Risk-of-commodification-of-SoHO-2022-11-18-Final.pdf/79e289b0-a02e-d3db-60c9-b9110ef704f2?t=1669631797556.
- Chen HI, Wolf JA, Blue R, Song MM, Moreno JD, Ming GL, Song H. Transplantation of Human Brain Organoids: Revisiting the Science and Ethics of Brain Chimeras. Cell Stem Cell. 2019 Oct 3;25(4):462-472. doi: 10.1016/j.stem.2019.09.002. PMID: 31585092; PMCID: PMC7180006.
- Hartung T, Morales Pantoja IE, Smirnova L. Brain organoids and organoid intelligence from ethical, legal, and social points of view. Front Artif Intell. 2024;6:1307613. Published 2024 Jan 5. Doi:10.3389/frai.2023.1307613.